INTRODUCTION
At the root of all science is our own unquenchable curiosity about ourselves and our world. We marvel, as our ancestors did thousands of years ago, when fireflies light up a summer evening. The colors and smells of nature bring subtle messages of infinite variety. Blindfolded, we know whether we are in a pine forest or near the seashore. We marvel. And we wonder. How does the firefly produce light? What are the substances that characterize the fragrance of the pine forest? What happens when the green leaves of summer are replaced by the red, orange, and gold of fall?
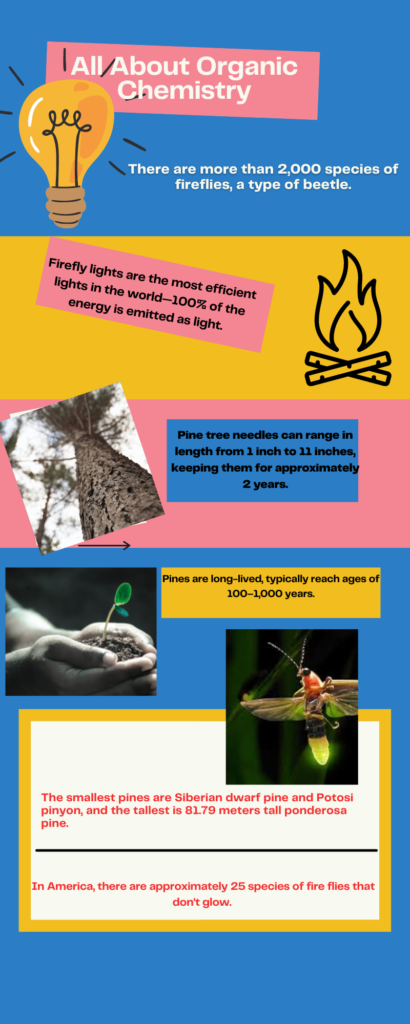
THE ORIGINS OF ORGANIC CHEMISTRY
As one of the tools that fostered an increased understanding of our world, the science of chemistry—the study of matter and the changes it undergoes—developed slowly until near the end of the eighteenth century. About that time, in connection with his studies of combustion the French nobleman Antoine Laurent Lavoisier provided the clues that showed how chemical compositions could be determined by identifying and measuring the amounts of water, carbon dioxide, and other materials produced when various substances were burned in air.
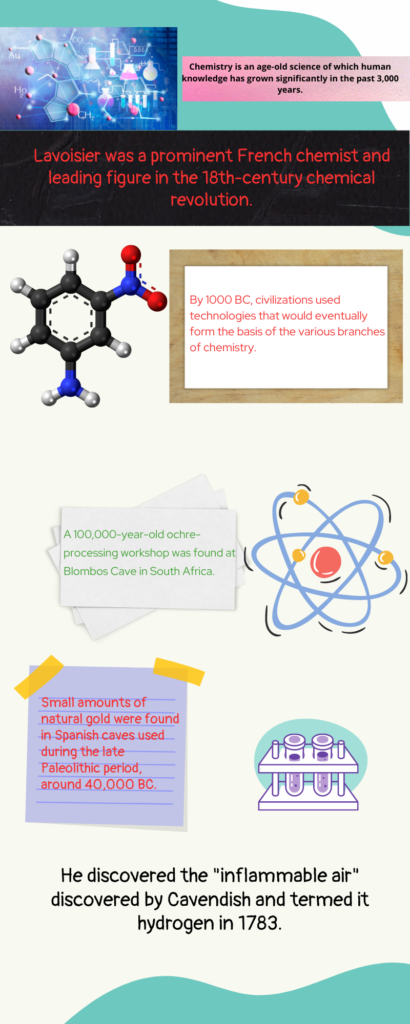
By the time of Lavoisier’s studies, two branches of chemistry were becoming recognized. One branch was concerned with matter obtained from natural or living sources and was called organic chemistry. The other branch dealt with substances derived from nonliving matter—minerals and the like. It was called inorganic chemistry.
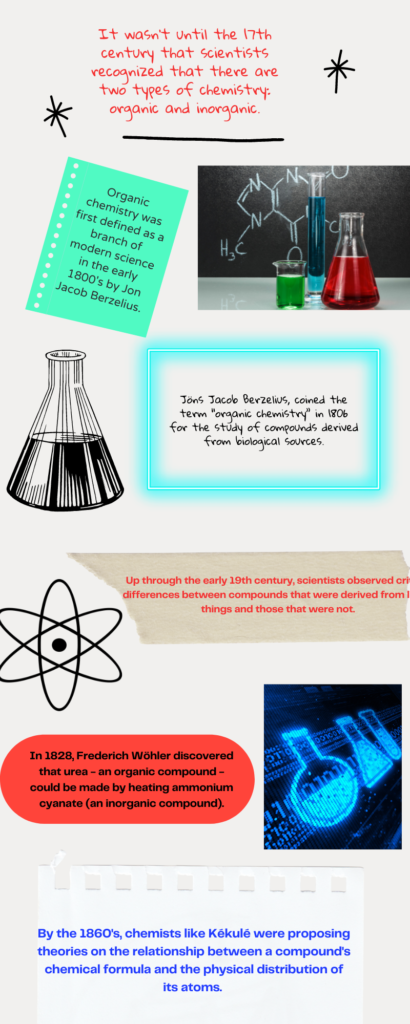
Combustion analysis soon established that the compounds derived from natural sources contained carbon, and eventually a new organic chemistry define emerged: organic chemistry define, is the study of carbon compounds. This is the definition we still use today
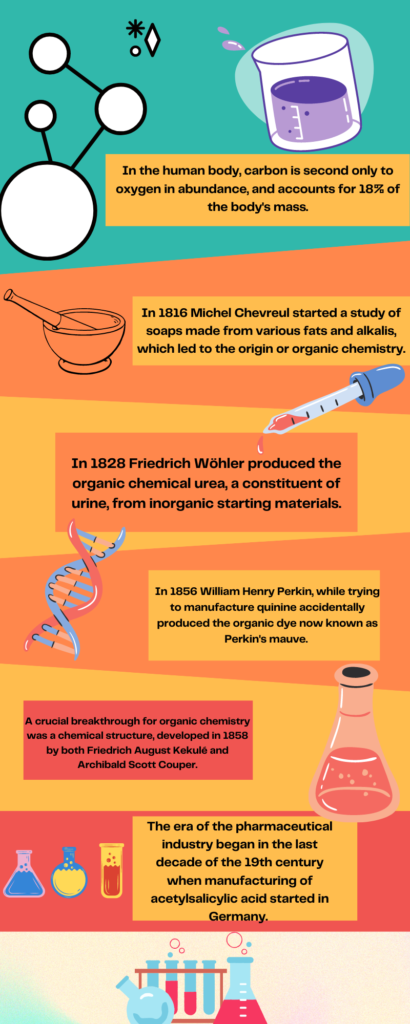
BERZELIUS, WÖHLER, AND VITALISM
As the eighteenth century gave way to the nineteenth, Jöns Jacob Berzelius emerged as one of the leading scientists of his generation. Berzelius, whose training was in medicine, had wide-ranging interests and made numerous contributions in diverse areas of chemistry.
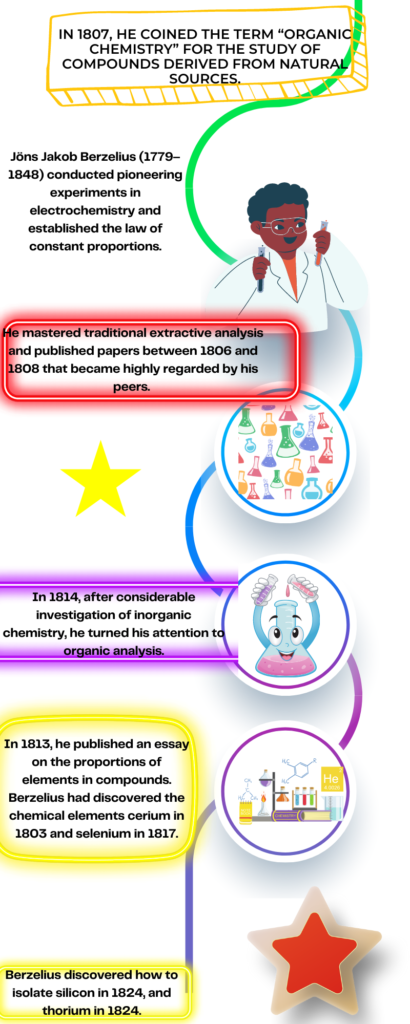
Berzelius, like almost everyone else at the time, subscribed to the doctrine known as vitalism. Vitalism held that living systems possessed a “vital force” which was absent in nonliving systems. Compounds derived from natural sources (organic) were thought to be fundamentally different from inorganic compounds; it was believed inorganic compounds could be synthesized in the laboratory, but organic compounds could not—at least not from inorganic materials
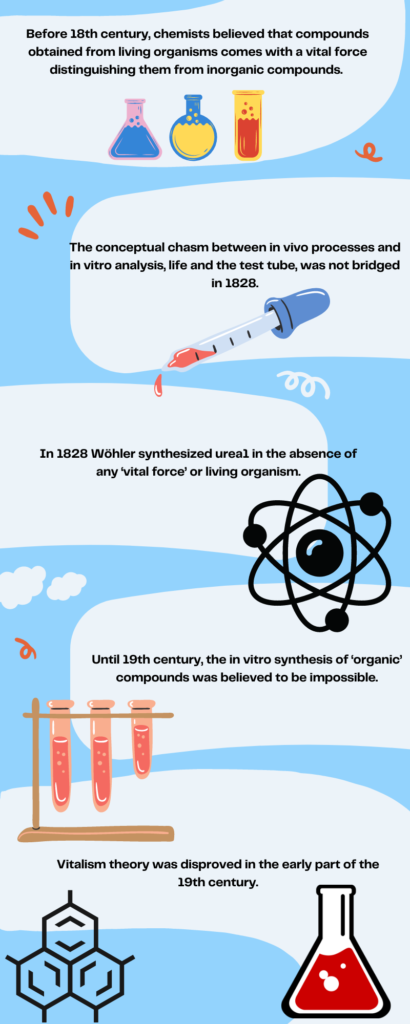
In 1823, Friedrich Wöhler, fresh from completing his medical studies in Germany, traveled to Stockholm to study under Berzelius. A year later Wöhler accepted a position teaching chemistry and conducting research in Berlin. He went on to have a distinguished career, spending most of it at the University of Göttingen, but is best remembered for a brief paper he published in 1828. Wöhler noted that when he evaporated an aqueous solution of ammonium cyanate, he obtained “colorless, clear crystals often more than an inch long,” which were not ammonium cyanate but were instead urea.
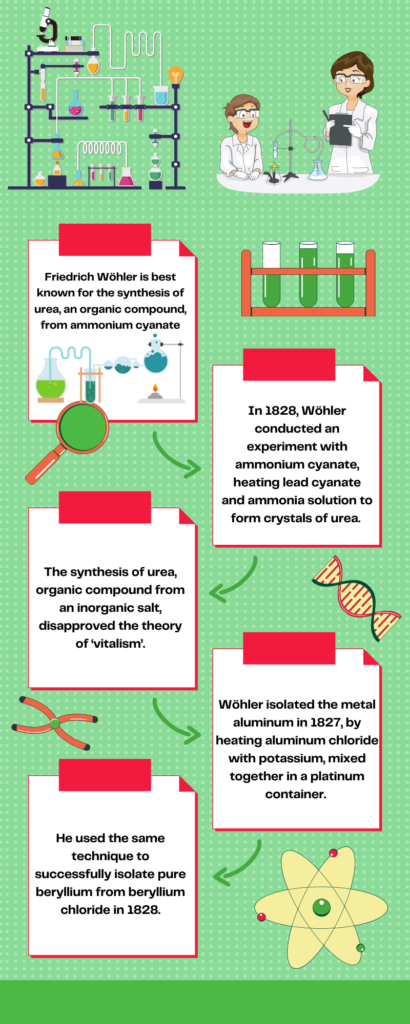
The transformation observed by Wöhler was one in which an inorganic salt, ammonium cyanate, was converted to urea, a known organic substance earlier isolated from urine. This experiment is now recognized as a scientific milestone, the first step toward overturning the philosophy of vitalism. Although Wöhler’s synthesis of an organic compound in the laboratory from inorganic starting materials struck at the foundation of vitalist dogma, vitalism was not displaced overnight. Wöhler made no extravagant claims concerning the relationship of his discovery to vitalist theory, but the die was cast, and over the next generation organic chemistry define outgrew vitalism.
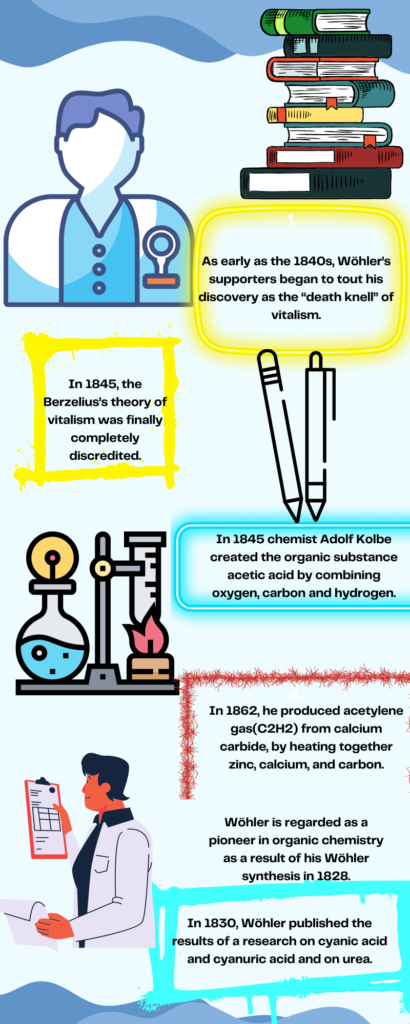
What particularly seemed to excite Wöhler and his mentor Berzelius about this experiment had very little to do with vitalism. Berzelius was interested in cases in which two clearly different materials had the same elemental composition, and he invented the term isomerism to define it. The fact that an inorganic compound (ammonium cyanate) of molecular formula CH4N2O could be transformed into an organic compound (urea) of the same molecular formula had an important bearing on the concept of isomerism.
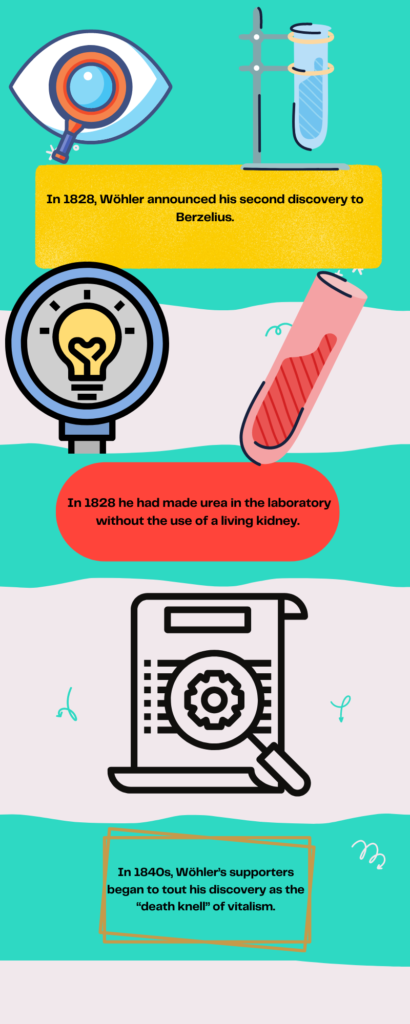
THE INFLUENCE OF ORGANIC CHEMISTRY
Many organic compounds were known to and used by ancient cultures. Almost every known human society has manufactured and used beverages containing ethyl alcohol and has observed the formation of acetic acid when wine was transformed into vinegar.
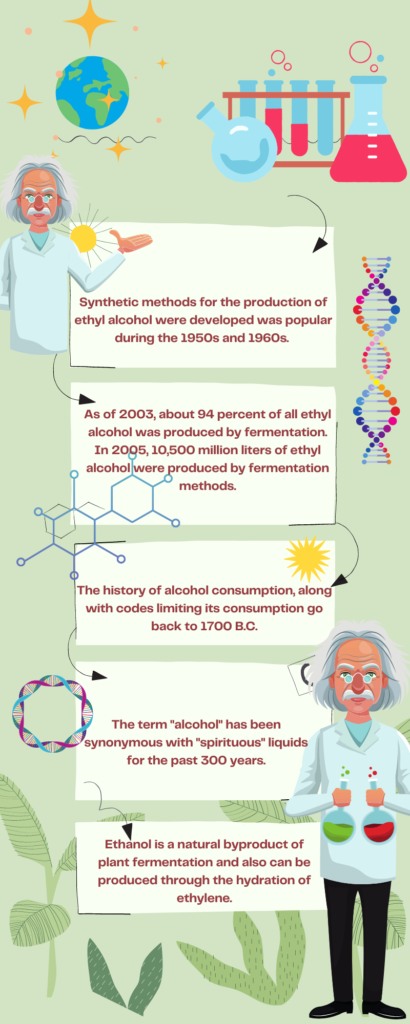
Early Chinese civilizations (2500–3000 BC) extensively used natural materials for treating illnesses and prepared a drug known as ma huang from herbal extracts. This drug was a stimulant and elevated blood pressure. We now know that it contains ephedrine, an organic compound similar in structure and physiological activity to adrenaline, a hormone secreted by the adrenal gland. Almost all drugs prescribed today for the treatment of disease are organic compounds—some are derived from natural sources; many others are the products of synthetic organic chemistry.

As early as 2500 BC in India, indigo was used to dye cloth a deep blue. The early Phoenicians discovered that a purple dye of great value, Tyrian purple, could be extracted from a Mediterranean Sea snail. The beauty of the color and its scarcity made purple the color of royalty. The availability of dyestuffs underwent an abrupt change in 1856 when William Henry Perkin, an 18-year-old student, accidentally discovered a simple way to prepare a deep-purple dye, which he called mauveine, from extracts of coal tar. This led to a search for other synthetic dyes and forged a permanent link between industry and chemical research
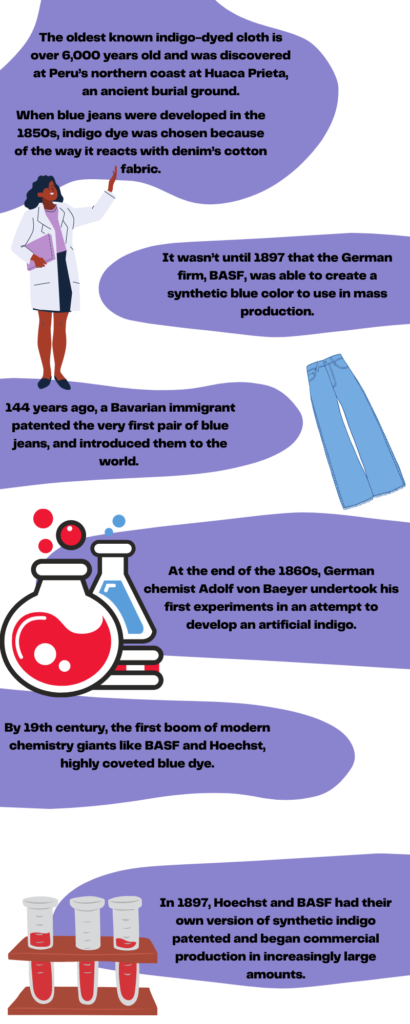
The synthetic fiber industry as we know it began in 1928 when E. I. Du Pont de Nemours & Company lured Professor Wallace H. Carothers from Harvard University to direct their research department. In a few years Carothers and his associates had produced nylon, the first synthetic fiber, and neoprene, a rubber substitute. Synthetic fibers and elastomers are both products of important contemporary industries, with an economic influence far beyond anything imaginable in the middle 1920s.
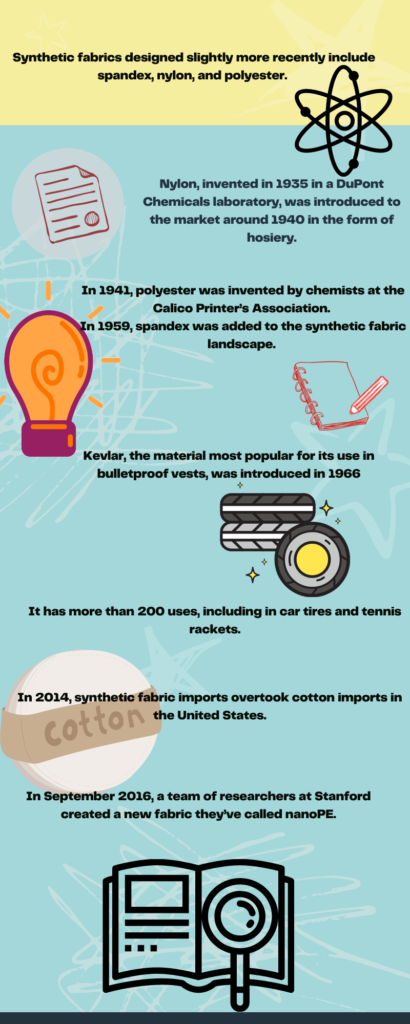
COMPUTERS AND ORGANIC CHEMISTRY
A familiar arrangement of the sciences place’s chemistry between physics, which is highly mathematical, and biology, which is highly descriptive. Among chemistry’s subdisciplines, organic chemistry is less mathematical than descriptive in that it emphasizes the qualitative aspects of molecular structure, reactions, and synthesis. The earliest applications of computers to chemistry took advantage of the “number crunching” power of mainframes to analyze data and to perform calculations concerned with the more quantitative aspects of bonding theory.
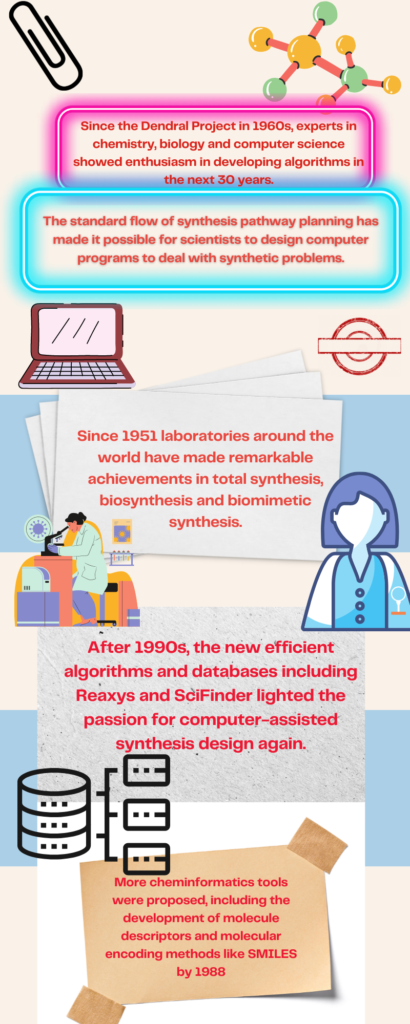
More recently, organic chemists have found the graphics capabilities of minicomputers, workstations, and personal computers to be well suited to visualizing a molecule as a three-dimensional object and assessing its ability to interact with another molecule. Given a biomolecule of known structure, a protein, for example, and a drug that acts on it, molecular-modeling software can evaluate the various ways in which the two may fit together.
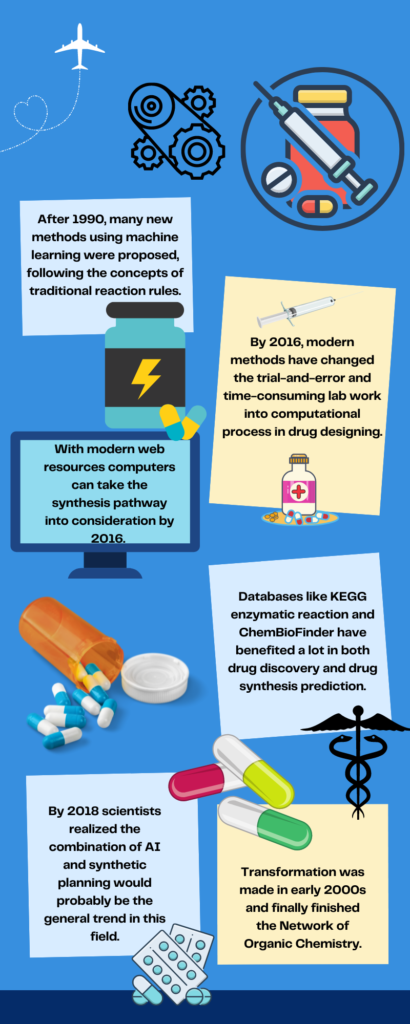
Such studies can provide information on the mechanism of drug action and guide the development of new drugs of greater efficacy. The influence of computers on the practice of organic chemistry is a significant recent development and will be revisited numerous times in the chapters that follow
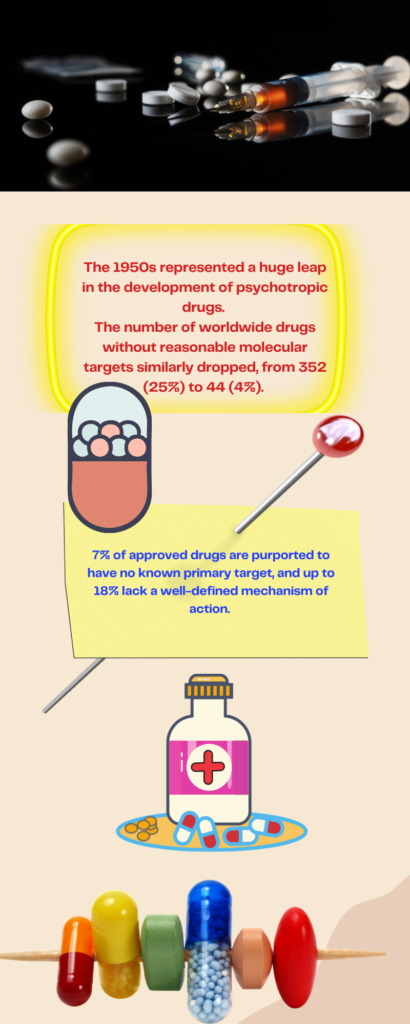
CHALLENGES AND OPPORTUNITIES
A major contributor to the growth of organic chemistry define, during this century has been the accessibility of cheap starting materials. Petroleum and natural gas provide the building blocks for the construction of larger molecules. From petrochemicals comes a dazzling array of materials that enrich our lives: many drugs, plastics, synthetic fibers, films, and elastomers are made from the organic chemicals obtained from petroleum.
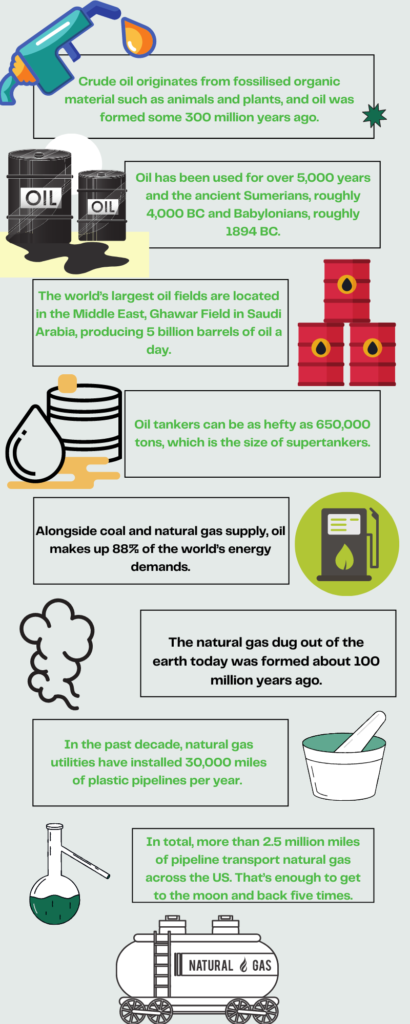
As we enter an age of inadequate and shrinking supplies, the use to which we put petroleum looms large in determining the kind of society we will have. Alternative sources of energy, especially for transportation, will allow a greater fraction of the limited petroleum available to be converted to petrochemicals instead of being burned in automobile engines. At a more fundamental level, scientists in the chemical industry are trying to devise ways to use carbon dioxide as a carbon source in the production of building block molecules.
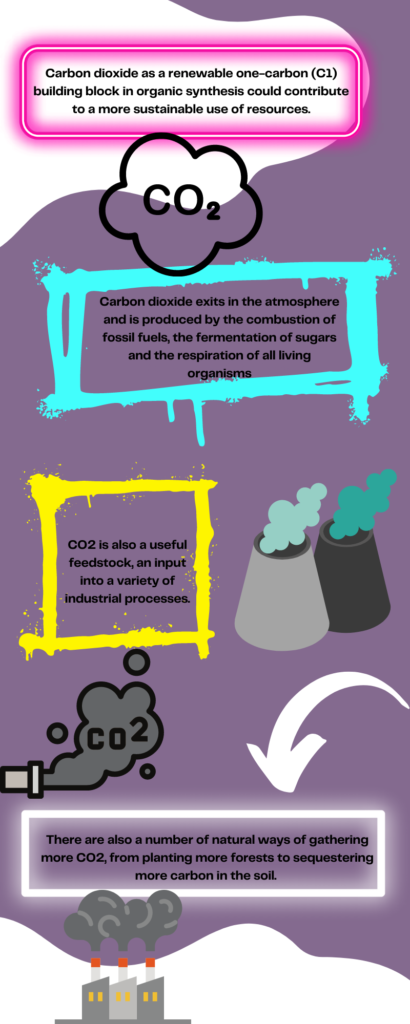
Many of the most important processes in the chemical industry are carried out in the presence of catalysts. Catalysts increase the rate of a particular chemical reaction but are not consumed during it. In searching for new catalysts, we can learn a great deal from biochemistry, the study of the chemical reactions that take place in living organisms. All these fundamental reactions are catalyzed by enzymes. Rate enhancements of several millionfold are common when one compares an enzyme-catalyzed reaction with the same reaction performed in its absence. Many diseases are the result of specific enzyme deficiencies that interfere with normal metabolism.
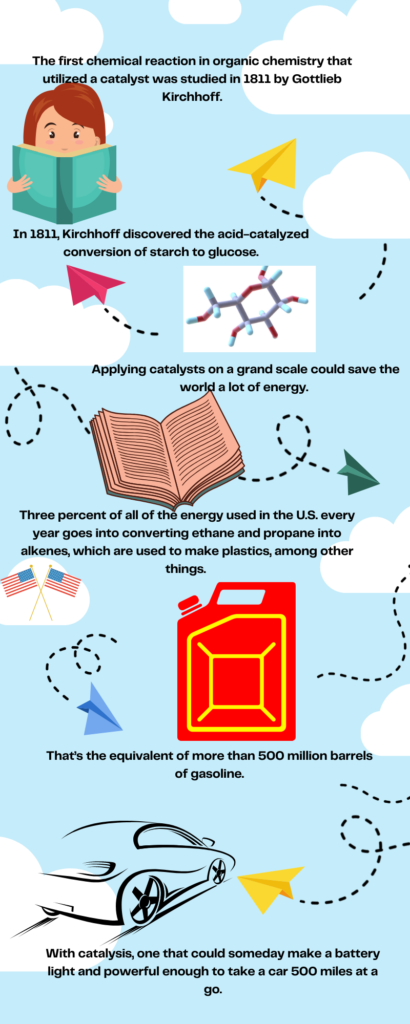
In the final analysis, effective treatment of diseases requires an understanding of biological processes at the molecular level—what the substrate is, what the product is, and the mechanism by which substrate is transformed to product. Enormous advances have been made in understanding biological processes. Because of the complexity of living systems, however, we have only scratched the surface of this fascinating field of study.
Spectacular strides have been made in genetics during the past few years. Although generally considered a branch of biology, genetics is increasingly being studied at the molecular level by scientists trained as chemists. Gene-splicing techniques and methods for determining the precise molecular structure of DNA are just two of the tools driving the next scientific revolution. You are studying organic chemistry define at a time of its greatest influence on our daily lives, at a time when it can be considered a mature science, when the challenging questions to which this knowledge can be applied have never been more important.